Dark matter is one of the most mysterious parts of the universe—unseen, yet pervading the cosmos, detectable only by its gravitational influence. Perhaps even stranger is that we’ve known it’s there since the 1970s, but we still don’t know what it is. Theories abound, but none of them have solid evidence.
When I was a grad student at Princeton, I saw a chart posted on the wall in the graduate lounge describing the state of the theory of dark matter in the 1980s. The Dark Matter Flowchart (link to Dr. Weinberg’s website) was created by three Princeton astrophysics grad students and one researcher circa 1986, describing a “meta-theory” of the general trend of new theories and papers on the subject. I thought it was hilarious, if dated, and even though it was written before I was born, it’s still surprisingly (and depressingly) as relevant as ever.
I’ve been wanting to write a post on the Flowchart for a while and explain all the references inside it. (I know, I know, I’m explaining the joke, but I still think the science is interesting.) The original Flowchart was printed on a TeX machine across three pages and is difficult to read, so I needed to transcribe it into something more modern. I ended up tracing it in GIMP and then adding a few…dozen footnotes. Some of the ideas listed in the flowchart are obsolete, but to someone familiar with cosmology and particle physics, much of it is still as relevant (and hilarious) as ever.
Special thanks to Drs. Weinberg and Ryden for answering some of my questions about the Flowchart, and to Dr. Lauer for his later feedback. So, without further ado, here is The Dark Matter Flowchart, Annotated.
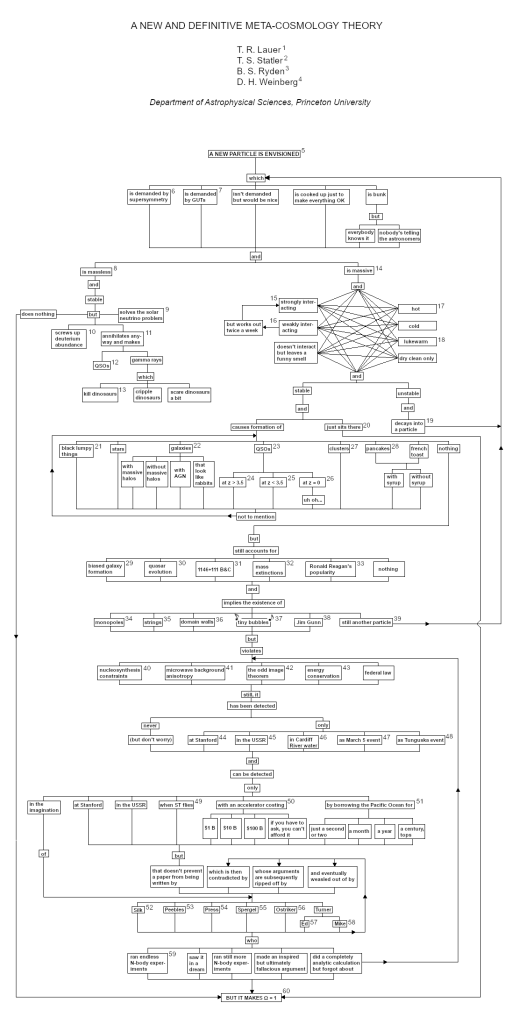
- T. R. Lauer
Dr. Tod Lauer received a Ph.D. from UC Santa Cruz in 1983. He was a postdoc at Princeton from 1983 to 1985, and remained there as a research staff member from 1985 to 1990. He studied elliptical galaxies at the time and later worked on the Hubble Space Telescope and the New Horizons mission to Pluto. He now works at the National Optical Astronomy Observatory (NOAO) in Tuscon, studying galaxies and the large-scale structure of the universe. He is also the only one of the four authors to have his own Wikipedia page. - T. S. Statler
Dr. Tom Statler received a Ph.D. from Princeton in 1986 for his work on modelling elliptical galaxies. He is now a Professor of Astronomy at the University of Maryland, mainly studying Near-Earth Asteroids. - B.S. Ryden
Dr. Barbara Ryden received a Ph.D. from Princeton in 1987 for her work on galaxy formation. She is now a Professor of Astronomy at The Ohio State University and has written several astrophysics textbooks. - D.H. Weinberg
Dr. David Weinberg received a Ph.D. from Princeton in 1989 for his work on the structure of the early universe. He is now the Chair of the Department of Astronomy at The Ohio State University and has done a lot of work with the Sloan Digital Sky Survey.
- A NEW PARTICLE IS ENVISIONED
This “theory” doesn’t say so directly, but then and now, this would usually mean a particle to explain dark matter. - Is demanded by supersymmetry.
Supersymmetry is a theory that tries to solve a lot of the problems in particle physics by postulating that every type of particle has a much heavier partner with specific properties. One of these particles would be a good for for dark matter. It looked very promising for a while, but unfortunately, in the past few years, the Large Hadron Collider has disproved the most likely versions of supersymmetry. - Is demanded by GUTs.
A GUT, or Grand Unified Theory, is any theory that explains the strong and weak nuclear forces as a single force. (This does not include gravity, which would make it a theory of everything.) Theories of cosmic inflation implicitly assume a GUT-like force, and sometimes include particles that would work as dark matter, but there are a lot of different versions of them, and they’re very hard to test. - Is massless.
A massless dark matter particle isn’t impossible. Dark matter needs mass, but massless particles still have energy, and Einstein told us energy is equivalent to mass. At the time, it was still believed that neutrinos were (or at least might be) massless, and that they could be dark matter. It was only later that cosmologists figured out that dark matter (probably) needs to be made of heavy, slow-moving particles to create galaxies as we see them. - Solves the solar neutrino problem.
The solar neutrino problem was that neutrino detectors saw about half as many neutrinos coming from the Sun as there should be. As early as 1968, it was suggested that if neutrinos had mass, they could change from one type to another in a process called neutrino oscillation. Since the detectors at the time could only see electron neutrinos, they were missing the ones that turned into muon neutrinos in transit. However, this was not proven until 2002, so it was still an open question at the time.
Edit: Dr. Lauer later informed me that this was a reference to Institute for Advanced Study director John Bahcall frequently talking at length about the problem. - Screws up deuterium abundance.
The amount of deuterium (heavy hydrogen) in the universe is very sensitive to conditions in the Big Bang and is one of the most useful tests of cosmological theories. If you do the math, and the deuterium abundance comes out wrong, your theory of dark matter is probably wrong. - Annihilates anyway and makes…
In the most popular theories of dark matter, dark matter particles are their own antiparticles, so when two meet, they can annihilate into more conventional particles. In most theories, this happens very slowly, but in some, it happens fast enough that you have to consider the new particles they create.
Edit: Dr. Lauer later informed me that this item was about scientists who would get “stuck” in the chart with their theory and would make an excuse about the particles annihilating to get out of it. I feel like a modern equivalent in my own field would be, “But the observations are blocked by clouds anyway.” - …QSOs
Short for Quasi-Stellar Objects, better known as quasars. Quasars are incredibly bright sources of radio waves (and other kinds of light) that were common in the early universe. We now know that they’re caused by huge amounts of gas falling into supermassive black holes, but at the time, we were only beginning to figure that out. - …gamma rays, which kill dinosaurs.
The Alvarez Hypothesis, that the dinosaurs were killed by an asteroid, is common knowledge now, but it wasn’t proposed until 1980, and other scientists were very skeptical of it until the Chicxulub Crater was discovered in 1990. Before that, many other theories were advanced to explain the extinction of the dinosaurs, including that Earth was irradiated by gamma rays from a nearby supernova. - Is massive.
“Massive” in this case means that the particle has any mass at all. So even the very light neutrinos as we know them today would fall into this category. - Strongly interacting.
This generally doesn’t refer to the strength of the interaction, but that it interacts with other particles by one of the two “strong” forces: the strong nuclear force and electromagnetism. This almost certainly isn’t true of dark matter, or else we would have seen it already. - Weakly interacting.
This means that the particle interacts only by the two “weak” forces, the weak nuclear force and gravity, making it much harder to see because it has much smaller effects on its surroundings. - Hot/Cold.
Just as with molecules, “hot” refers to how fast dark matter particles are moving. Lighter particles (like neutrinos) are usually hotter. This has observable effects on galaxy formation, and we now know that a large fraction of dark matter must be cold to match what we see. - Lukewarm.
No, there’s no theory of “lukewarm dark matter,” but more recently, there is a new theory of “warm dark matter.” If there is an especially heavy fourth kind of neutrino, it might qualify. - Is unstable and decays into a particle…
Oops, back to start. Maybe your theory demands this particle, but it won’t solve your problem if it decays into something else first. (Although this would also apply to the annihilating part above.) - Is stable and…
…just sits there.
This is starting to look worryingly plausible for real dark matter considering how hard it is to find.
…causes the formation of…
- …black lumpy things.
I checked with Dr. Weinberg and confirmed that this is referring to MACHOs, or Massive Compact Halo Objects, which are collapsed stars or lumps of normal matter than don’t give off light and therefore are technically a form of dark matter. However, studies of gravitational lensing have proven that there are just not that many MACHOs around. - …galaxies…
There are a lot of different moving parts to galaxy formation that have to be accounted for, including active galactic nuclei (AGN) and dark matter halos, and we’re still trying to figure it out. - …QSOs…
Again. Interestingly, we now know that AGN and QSOs are the same thing. - …at z>3.5.
These are high-redshift objects from the early universe. We see lots of them. - …at z<3.5.
These are less redshifted objects, but still from the young universe. We see lots of these, too. - …at z=0. Uh-oh…
z=0 means a redshift of zero, which means objects in the nearby universe and the present day. The nearest quasar is 3C 273, which is 1.7 billion light-years away at a redshift of z=0.158, so if your theory predicts they should be nearby, you have a problem. - …clusters.
Clusters of galaxies have many of the same problems as galaxy formation. - …pancakes.
Believe it or not, this is a real scientific term. (Hence why including French toast as a joke is funny.) Zeldovich Pancakes were theoretical objects created by hot dark matter theories, in which huge, supercluster-sized clouds of gas would collapse into flattened, pancake shapes and then break up into smaller and smaller chunks until they formed galaxies. Unfortunately, this model formed galaxies too slowly, so cosmologists switched to cold dark matter theories that form them faster.
…nothing, but still accounts for…
- …biased galaxy formation.
Biased galaxy formation is a model where galaxies only form in areas where dark matter is especially dense. This is necessary to account for the amount of dark matter that seems to be out there, but at the time, it was poorly understood, so they had to manually go in and adjust it in the computer simulations. Now, we know that there is less dark matter than we thought, and we understand why it happens better. The underlying “bias” toward galaxies forming around denser dark matter is still there, however, and it appears to be even more true for dwarf galaxies. - …quasar evolution.
Quasars change in brightness very quickly, on the scale of weeks. This is surprising because it means that they have to be smaller than a few light-weeks in size, and it’s hard to pack that much brightness into such a small space. With the supermassive black hole model, however, it’s easy to explain. - 1146+111 B&C.
These are two quasars that appear very close together in the sky. For a while, we thought they might be two images of the same quasar caused by gravitational lensing, but it turned out to just be a coincidence. - Mass extinctions.
Again. - Ronald Reagan’s popularity.
This joke would be even better with Donald Trump today.
And implies the existence of…
- …monopoles.
Magnetic monopoles are particles that have a “magnetic charge,” meaning a single magnetic pole, whereas all normal magnets have two. They’re predicted by many Grand Unified Theories and cosmic inflation, but they’re also predicted to be extremely rare. They also form in a weird way that makes them a sort of point-defect in spacetime rather than quantum field oscillations like other particles. - …strings.
Just as monopoles are zero-dimensional defects in spacetime, cosmic strings are one-dimensional defects predicted by the same theories. They have enormous mass per unit length, almost like a singularity, and they bend space around them, but they would also be very rare. - …domain walls.
These are two-dimensional defects in spacetime. Also very rare. - …Tiny Bubbles.
A song by Don Ho (1966). However, Dr. Ryden says that this was a play on “big bubbles” produced by the “explosive galaxy formation” model of Ostriker & Cowie (1981). These big bubbles are better known as voids today. Voids are regions of space that can be up to a hundred million light-years across where there are almost no galaxies. Explosive galaxy formation theorizes that a bunch of supernovae exploding in an especially early-forming galaxy at (relatively) the same time could blow these bubbles into intergalactic space. However, even a very large number of supernovae probably don’t have enough energy to do this. Today, we know that the way dark matter clumps together can explain these voids on its own.
Interestingly, today, there are models of cosmic inflation with “bubble universes,” where bubbles of “normal” space appear spontaneously out of an eternally inflating spacetime. - …Jim Gunn.
James Edward Gunn received a Ph.D. from Caltech in 1965. At the time, he was a Professor of Astrophysics at Princeton, and he remains there today as Emeritus. Dr. Gunn is especially known for co-predicting the existence of the Gunn-Peterson trough, a spectral feature of distant quasars, in 1965, which was not seen in nature until 2001. Not to be confused with award-winning science fiction author James Edwin Gunn, Guardians of the Galaxy director James Francis Gunn, Jr., or Chicago Bears linebacker Jimmy Gunn. - …still another particle.
And you’re back to the start again.
But violates…
- …nucleosynthesis constraints.
This is the same problem with deuterium from earlier. - …microwave background anisotropy.
The cosmic microwave background (CMB) looks almost exactly the same in every direction. Any deviation from this uniform appearance is called an “anisotropy.” At the time, anisotropy was theoretical. There were no space-based measurements of the CMB, and the only irregularity we’d found was a slight difference between one side of the sky and the other caused by the motion of our solar system. We now know that there is very complex pattern of anisotropies, but they are very small differences, and the overall pattern is still the same in every direction. If your theory predicts the CMB should be different in different directions, you have a problem. - …the odd image theorem.
Gravitational lensing, the bending of light by gravity as it moves through space, can cause multiple images of a distant object like a quasar to appear in different places on the sky. The Odd Image Theorem says that there will always be an odd number of these images, because of the way the mathematics of lensing works. You won’t always see an odd number, though, because some images may be too faint to see or may be obscured by another object. - …energy conservation.
Ironically, general relativity doesn’t conserve energy to begin with. Photons lose energy as space expands because their wavelengths get stretched out. And the amount of dark energy in the universe increases as the amount of space increases. A complicated theorem called Noether’s Theorem says that conservation of energy only applies when the properties of the universe are constant in time, and since it’s expanding, they’re not constant.
Still, it has been detected only…
- …at Stanford.
The Stanford Linear Accelerator is a powerful particle accelerator, but even at the time, it wasn’t necessarily where you would expect to find new physics. At the time, the most powerful particle accelerator in the world—the one that had already discovered the W and Z bosons—was the Super Proton Synchrotron at CERN—what was later renovated into the Large Hadron Collider. Edit: the Large Hadron Collider was actually a replacement for the Large Electron Positron Collider, not the smaller SPS. I was confused by this, but Dr. Weinberg says that this was a reference to an experiment by Stanford physicist Blas Cabrera Navarro (not to be confused with his grandfather, physicist Blas Cabrera Felipe), who claimed to have detected magnetic monopoles there (see above). If the chart were written today, he says, this might read “under an Italian Alp,” referring to the infamous “faster than light neutrinos” experiment. - …in the USSR.
Laboratories in the Soviet Union were also known for powerful particle accelerators, especially the kind that produced new elements. However, the Soviet Union in general also had a reputation for overstating its achievements. - …in Cardiff river water.
This one really stumped me, but Dr. Weinberg confirmed that it does indeed refer to the only reference I can find to “Cardiff river water” in the literature. In this paper, Fred Hoyle and Chandra Wickramasinghe claimed that molecules found in the exoskeletons of microorganisms in Cardiff river water were also found in space in the Trapezium Nebula (better known as the Orion Nebula). This was purported to show that life on Earth came from space through a process known as panspermia.
Fred Hoyle was a highly-renowned astronomer who had helped discover the nuclear processes inside stars, but also had some eccentric ideas like panspermia and disbelieving the Big Bang theory. (He actually coined the term “Big Bang” as a way of mocking the theory.) His student, Chandra Wickramasinghe, still promotes panspermia today, along with even more fringe ideas like some diseases coming from outer space. - …as March 5 event.
On March 5, 1979, multiple satellites observed a gamma ray burst 100 times more powerful than anything ever recorded at the time. The cause of it was not explained until 1992, but is now known to have been a starquake on a magnetar—that is, an extremely powerful gamma ray flare on a neutron star with an unusually strong magnetic field. This kind of object is now called a soft gamma repeater. - …as Tunguska event.
On June 30, 1908, a meteor crashed near the Tunguska River in Siberia, causing an explosion so powerful that it leveled 800 square miles of forest (2,000 square kilometers). The impact was thought to have been caused by a comet as early as the 1930s, but in the 1980s, there was debate over whether it was a comet or an asteroid. The Tunguska event is also a favorite target for fringe theories like mini-black holes and space aliens.
And can be detected only…
- …when ST flies.
ST stands for Space Telescope, which is what the Hubble Space Telescope was called before it got a proper name. Originally proposed to launch in 1983, technical delays, budget problems, the Space Shuttle Challenger exploding, and a mistake in the design of the mirror meant that it wasn’t usable until 1993. Sadly, the upcoming James Webb Space Telescope hasn’t fared any better. - …with an accelerator costing $1 billion / $10 billion / $100 billion / if you have to ask, you can’t afford it.
The original chart wrote the billions with exponents, which is probably funnier, but less readable. These numbers were high then and to some extent are high even today. After all, even the Large Hadron Collider cost less than $10 billion to build. On the other hand…times have changed. In 1985, the federal budget deficit was $212 billion, and that was considered high. In 2018, it was $779 billion, and it’s been over a trillion in recent years. - …by borrowing the Pacific Ocean for just a second or two / a month / a year / a century, tops.
Detectors for weakly interacting particles like neutrinos or dark matter often work by storing a large tank of water or some other substance deep underground. When a particle hits a water molecule, it produces a tiny flash of light that sensitive electronics can pick up. It has to be deep underground because only weakly-interacting particles can penetrate that far, while all other forms of radiation are blocked by the rock. Over the years, detectors keep getting bigger and bigger, and we’ve gotten pretty good at detecting neutrinos, but we still haven’t seen any dark matter. The largest neutrino detector in the world, IceCube, uses natural water ice, with electronics drilled into a billion tons of ice at the South Pole, and even it hasn’t seen any dark matter, although it isn’t sensitive to all possible versions of it.
But that doesn’t prevent a paper from being written by…
- Silk
Dr. Joseph Silk received a Ph.D. from Harvard in 1968. At the time, he was a Professor of Astronomy at UC Berkeley, and he is now an Emeritus Fellow at Oxford, among other things. Dr. Silk is especially known for predicting Silk damping (also known as diffusion damping) in 1968, a phenomenon that increases the smoothness of the universe on (relatively) small scales and was first observed in 2001. - Peebles
Dr. Jim Peebles received a Ph.D from Princeton in 1962. At the time, he was a Professor of Physics at Princeton, and he remains there now as Emeritus. Dr. Peebles is one of the recipients of the 2019 Nobel Prize in Physics for his overall body of work in cosmology. He was also one of the people who predicted the existence of the CMB. - Press
Dr. William H. Press received a Ph.D. from Caltech in 1973. At the time, he was a Professor of Astronomy at Harvard. Among his other credits, he later served as the deputy director of Los Alamos National Laboratory and as Vice Chair of President Obama’s Council of Science Advisers. Unusually, Dr. Press changed fields in 2007 and now works as a Professor of Computer Science and Integrative Biology at UT Austin. He also coauthored Numerical Recipes, a famous computer programming reference book, and he is known for the Press-Schechter formalism, a mathematical model for the distribution of masses of galaxies and galaxy clusters in the universe. Not to be confused with talk radio host Bill Press. - Spergel
Dr. David Spergel received a Ph.D. from Harvard in 1985, making him, unusually, a contemporary of the authors. At the time, he was a member of the Institute for Advanced Study in Princeton. He later moved over to the university, and he is now a Professor Emeritus of Astrophysics at Princeton and the Director of the Flatiron Institute’s Center for Computational Astrophysics in New York City. Dr. Spergel is especially known for his work on the WMAP space probe studying the CMB. - Ostriker
Dr. Jeremiah “Jerry” Ostriker received a Ph.D. from the University of Chicago in 1964. At the time, he was a Professor of Astrophysics at Princeton, and he remains there now as Emeritus. Along with Jim Peebles, Dr. Ostriker devised the Ostriker-Peebles criterion, which describes the circumstances in which a spiral galaxy will develop a central bar, and he contributed to the founding of the Sloan Digital Sky Survey. - Ed Turner
Dr. Edwin L. Turner received a Ph.D. from Caltech in 1975. At the time, he was a Professor of Astrophysics at Princeton, and he remains there now as Emeritus. Dr. Turner has studied a wide range of topics in cosmology and astrobiology. Sadly, he is the only one of these esteemed figures not to have his own Wikipedia page. Not to be confused with CNN founder Ted Turner. - Mike Turner
Dr. Michael S. Turner received a Ph.D. from Stanford in 1978. Then and now, he is a Professor of Physics and Astronomy at the University of Chicago. Dr. Turner is best known for coining the term “dark energy” in 1998. Not to be confused with Ohio Congressman Michael R. Turner.
- Who ran endless N-body experiments.
N-body experiments are computer simulations that model the behavior of large numbers of particles under the influence of gravity (and sometimes other forces). They’re tricky to do accurately and need a lot of computing power, so you often have to use workarounds like letting one particle represent many stars.
60. BUT IT MAKES Ω = 1
Ω, sometimes written as Ω_0 or Ω_tot, is the symbol for the total density of matter and energy in the universe. If it equals exactly 1, the universe is geometrically flat. That means parallel lines never meet, and the angles of a triangle add up to 180 degrees. With more or less matter, space would be curved, and those things wouldn’t happen. Also, those are things we can measure on the sky to within 1%, and they say that Ω=1. The problem is that when you add up all the matter and energy we can see, you only get Ω=0.05, which is why we’re looking for new particles in the first place. At the time, it was believed that the other 95% of the universe was all dark matter, but in 1998, dark energy was discovered. Dark energy probably isn’t a particle, but is mostly likely an intrinsic property of space-time, also known as a scalar field. In any case, we now believe that the universe is 26% dark matter and 69% dark energy, and all of these theories we’ve discussed are trying to explain that fact regardless of any other problems they cause.
For one final note, the following comment was added much later by Dr. Stacy McGaugh of Case Western Reserve university:
When this flowchart was written, it was known that Omega=1, Lambda=0 [dark energy], and H_0=50. Now it is known (with the same high confidence by the same perpetrators) that Omega=0.27, Lambda=0.73, and H_0=72. The situation described by the flowchart has changed rather less in that time.